I. Introduction
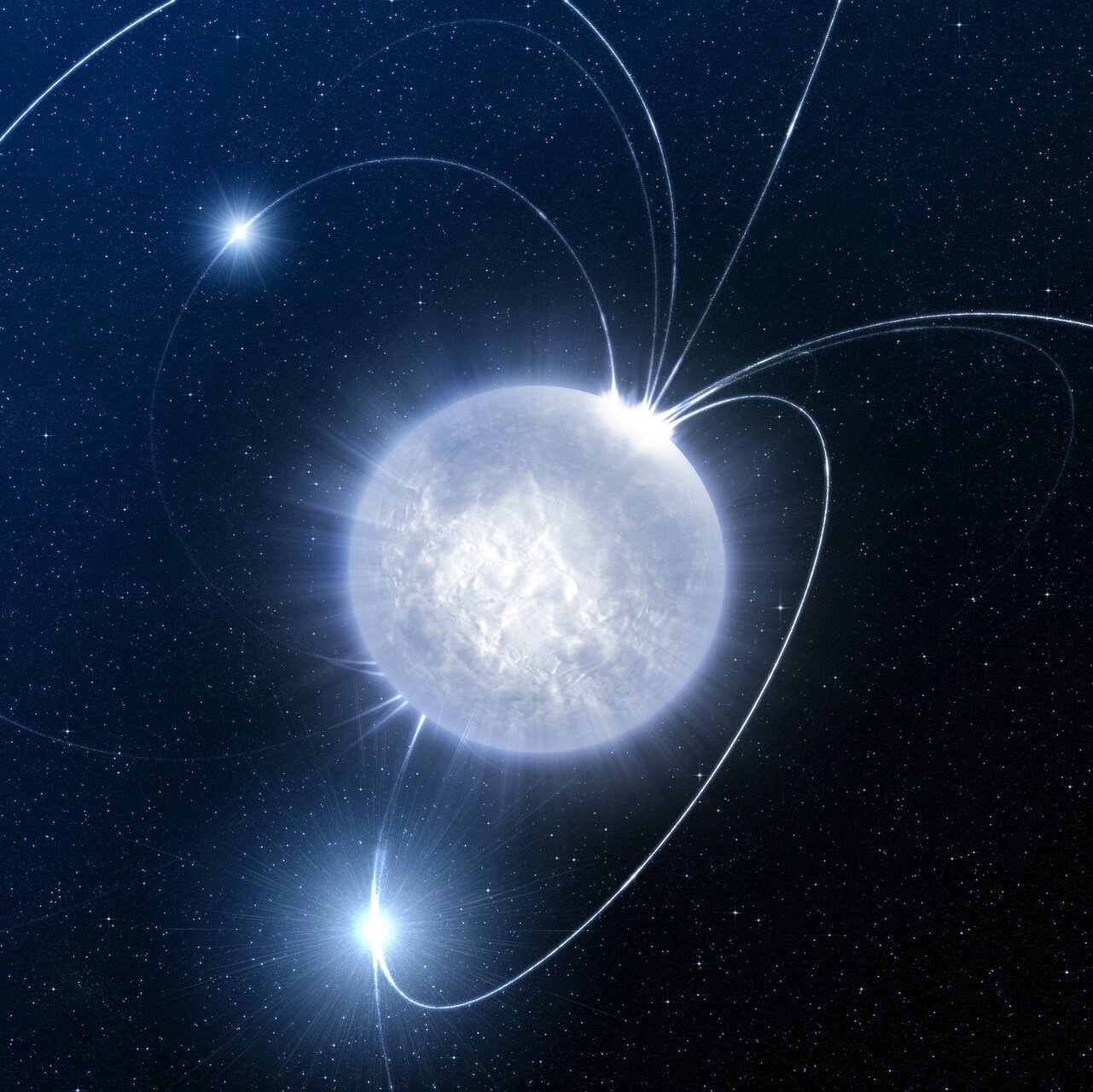
A. What are Magnetars and Why They Matter?
Magnetars are a special class of neutron stars that have incredibly strong magnetic fields. These fields are trillions of times stronger than the magnetic field of Earth and can create some of the most energetic phenomena in the universe.
B. Exploring the Significance of Studying Magnetars 🌟
Studying magnetars is important because they can provide us with valuable information about the extreme physics that exist in the universe. By studying these highly magnetized objects, we can learn more about how magnetic fields and high-energy particles interact in extreme environments.
C. The Fascinating History of Magnetar Discovery
The first magnetar was discovered in 1979 by a team of astronomers who were studying data from a series of X-ray bursts observed by the Ariel V satellite. They noticed that the bursts had properties that were different from anything they had seen before and later identified the source as a magnetar. Since then, over two dozen magnetars have been identified.
II. What are magnetars?
🌟 A. Defining Magnetars: The Most Magnetic Stars in the Universe
Magnetars are neutron stars with extremely strong magnetic fields, which are typically around 1,000 times stronger than those of regular neutron stars. These magnetic fields are so strong that they can cause the star’s crust to crack and create intense bursts of radiation.
🌟 B. Origins of Magnetars: How These Unique Stars are Formed?
Magnetars are formed when a massive star undergoes a supernova explosion, which leaves behind a dense, highly magnetized neutron star. The magnetic field of the star is then amplified through a process known as dynamo action, which is caused by the rotation of the star’s core.
🌟 C. Characteristics of magnetars
- Strong magnetic fields: Magnetars have the strongest magnetic fields of any known object in the universe, with some estimates putting their field strengths at around 10^15 Gauss.
- High rotational speeds: Magnetars can rotate at incredibly high speeds, with some spinning hundreds of times per second.
- Intense X-ray and gamma-ray emissions: Magnetars emit intense bursts of X-rays and gamma-rays, which are caused by the cracking of the star’s crust.
- Age and lifespan: Magnetars have relatively short lifespans, with most only existing for a few million years before their magnetic fields weaken and they become regular neutron stars.
III. Magnetars and their effects
🪐 A. The Impact on Their Surroundings
- Gamma-ray bursts: Magnetars have been observed to produce some of the brightest and most energetic explosions in the universe – known as gamma-ray bursts. These bursts release a huge amount of energy in the form of gamma rays and can last anywhere from a few milliseconds to several minutes.
- Electromagnetic pulses: Magnetars also emit intense bursts of electromagnetic radiation, known as giant flares, which can be millions of times more powerful than any observed solar flare. These pulses can disrupt electronic devices and affect communication systems.
- Magnetospheres: Magnetars have extremely strong magnetic fields, which can have a significant impact on their surroundings. The magnetic field can cause particles in the magnetosphere to accelerate to very high speeds, producing radiation and particles that can be observed by telescopes.
🌍 B. Effects of Magnetars on Earth
- Possible impact on climate: The intense bursts of electromagnetic radiation from magnetars can potentially have an impact on Earth’s climate. Some studies have suggested that gamma-ray bursts could cause ozone depletion and alter atmospheric chemistry.
- Radiation exposure: The intense radiation from magnetars could pose a risk to spacecraft and astronauts in space. This radiation can damage electronic equipment and cause radiation sickness in humans.
- Impact on satellite and communication systems: The electromagnetic pulses from magnetars can interfere with satellites and communication systems, causing disruptions and potentially damaging equipment. In addition, the strong magnetic fields can cause charged particles to become trapped in the Earth’s magnetic field, leading to increased radiation exposure for satellites in orbit.
IV. Differences between magnetars, black holes, and pulsars
Magnetars, black holes, and pulsars are all fascinating astronomical objects that exhibit unique properties. While all three objects are related to the collapse of massive stars, they differ in several key aspects.
A. Key differences between magnetars, black holes, and pulsars
- Formation: Magnetars and pulsars are formed when a massive star undergoes a supernova explosion and leaves behind a highly magnetized neutron star, while black holes are formed when the core of a massive star collapses under its own gravity.
- Magnetic fields: Magnetars are known for having the strongest magnetic fields in the universe, with magnetic fields that can be trillions of times stronger than that of the Earth. Pulsars also have strong magnetic fields, but they are typically much weaker than those of magnetars. In contrast, black holes do not have a magnetic field.
- Rotation: Magnetars and pulsars are known for their fast rotation, with some pulsars rotating hundreds of times per second. Black holes do not have a rotation, but instead, they have an event horizon, which is the point of no return beyond which nothing can escape.
- Emissions: Magnetars are known for their intense emissions of X-rays and gamma rays, which are produced as a result of their strong magnetic fields. Pulsars also emit X-rays, as well as radio waves and visible light. Black holes do not emit any radiation, but their presence can be detected through their effects on nearby matter.
- Lifespan: Magnetars and pulsars have finite lifespans, with magnetars expected to last for several thousand years, and pulsars for several million years. Black holes, on the other hand, have an almost infinite lifespan, as they do not decay over time.
B. Is a magnetar stronger than a black hole?
While magnetars have the strongest magnetic fields in the universe, they are not stronger than black holes in terms of their gravitational pull. Black holes are known for their extreme gravity, which is so strong that not even light can escape from their event horizon.
C. Are magnetars stronger than pulsars?
Magnetars are generally considered to be stronger than pulsars in terms of their magnetic fields and emissions. However, pulsars can still be incredibly powerful, with some emitting more energy than the Sun in a single second.
V. Nearest Magnetar to Earth 🌍💫
A. The Closest Magnetar to Earth: An Exploration🌟
The closest known magnetar to Earth is known as SGR 1806-20, located about 50,000 light-years away in the constellation Sagittarius. It was first discovered in 1979 when it emitted a burst of gamma rays that lasted only a fraction of a second. It was later identified as a magnetar, a type of neutron star with an incredibly strong magnetic field.
B. Importance of Studying the Nearest Magnetar 🧐
Studying SGR 1806-20 is important because it allows scientists to gain insights into the nature of magnetars and their potential effects on Earth. Because of its relative proximity to us, it is one of the few magnetars that can be studied in detail.
C. Characteristics of the Nearest Magnetar 🌟
- Location and Distance 🌠: SGR 1806-20 is located in the Milky Way galaxy, in the direction of the galactic center. It is approximately 50,000 light-years away from Earth.
- Emissions 🔥: The emissions from SGR 1806-20 are highly energetic, primarily in the form of X-rays and gamma rays. It has been known to emit giant flares, some of which have been among the most energetic events ever observed in the universe.
- Effects on Earth 🌍: While SGR 1806-20 is relatively far from Earth, its emissions can still have an impact on our planet. The strong magnetic fields associated with magnetars can cause disruptions to satellite and communication systems. In 2004, a giant flare from SGR 1806-20 was detected, and it caused a temporary ionospheric disturbance on Earth.
VI. Observing Magnetars: Tools and Techniques🌟🔭
A. How are magnetars observed? 👀
Magnetars are primarily observed through the detection of their high-energy X-ray and gamma-ray emissions, which are produced by the interaction of their strong magnetic fields with their surroundings. These emissions are typically detected using X-ray telescopes, such as NASA’s Chandra X-ray Observatory, and gamma-ray telescopes, such as NASA’s Fermi Gamma-ray Space Telescope.
B. Types of observations 🔍
- X-ray telescopes 🌟: X-ray telescopes are the primary tool for observing magnetars, as they are the best way to detect their X-ray emissions. These telescopes work by focusing X-rays onto a detector, which can then be used to create images of the magnetar’s emissions. Some of the X-ray telescopes that have been used to observe magnetars include NASA’s Chandra X-ray Observatory and the European Space Agency’s XMM-Newton telescope.
- Radio telescopes 📡: Radio telescopes can also be used to observe magnetars, although they are less sensitive to their emissions than X-ray telescopes. Radio telescopes work by detecting the radio waves emitted by magnetars, which can be used to study their magnetic fields and plasma environments. Some of the radio telescopes that have been used to observe magnetars include the Green Bank Telescope in West Virginia and the Parkes Radio Telescope in Australia.
- Optical telescopes 🌌: Optical telescopes are generally not used to observe magnetars, as they are not visible in the optical spectrum. However, they can be used to observe the magnetar’s environment and the effects of its emissions on nearby stars and gas clouds. Some of the optical telescopes that have been used to study magnetars include the Hubble Space Telescope and the Keck Observatory in Hawaii.
ALSO READ: The World of the Mysterious and Elusive Planet 9
VII. Advancements in Magnetar Research
A. Current Research Projects: Investigating Magnetars🧪
Currently, there are several ongoing research projects that are aimed at gaining a better understanding of magnetars. One such project is the Magnetar Outburst Rapid Response Experiment (MORRE), which is designed to quickly detect and study the outbursts of magnetars using a global network of telescopes. Another project, the Neutron star Interior Composition Explorer (NICER), is focused on studying the interiors of neutron stars, including magnetars, through X-ray observations.
B. Future Research Directions: Exploring the Unknown🔭
In the future, researchers are looking to further advance our understanding of magnetars by studying their magnetic fields, rotation rates, and the particles that they emit. One area of particular interest is the study of magnetar flares, which are sudden, intense bursts of energy that can release more energy in a few seconds than the Sun does in years. By studying these flares, researchers hope to gain insight into the extreme physics that governs these enigmatic objects.
There is also ongoing research to explore the relationship between magnetars and other astrophysical phenomena, such as gamma-ray bursts and fast radio bursts. Additionally, future research will focus on developing new observational techniques and tools to study magnetars, including next-generation X-ray and radio telescopes.
VIII. Magnetars and Astrophysics 🪐🧲🌌
A. Magnetars: Providing Unique Insights into Astrophysics🌟
Magnetars are important celestial objects for astrophysical studies because they provide a unique opportunity to study the most extreme conditions of matter, energy, and magnetic fields in the universe. The study of magnetars can help us better understand the physics of dense matter, strong magnetic fields, and high-energy astrophysical phenomena.
B. Magnetars as Laboratories for Extreme Physics 🧪
Magnetars can be thought of as natural laboratories for extreme physics. The incredibly strong magnetic fields of magnetars, which can be trillions of times stronger than the Earth’s magnetic field, can provide insights into the behavior of matter under such extreme conditions.
One area of research involving magnetars is the study of quantum electrodynamics (QED), the theory that describes the interaction between matter and electromagnetic fields. The strong magnetic fields of magnetars can create conditions where QED effects become significant, allowing researchers to study the behavior of particles in extreme conditions that cannot be replicated in Earth-based laboratories.
Another area of research involving magnetars is the study of high-energy astrophysical phenomena such as gamma-ray bursts and fast radio bursts. The intense X-ray and gamma-ray emissions from magnetars can provide insights into the mechanisms behind these mysterious events and help us better understand the origins of the universe.
IX. Magnetars vs. Black Holes?
🕳️ A. Exploring the Mechanics of Black Holes
A black hole is a region of space where gravity is so strong that nothing, not even light, can escape its grasp. This happens when a massive star dies and its core collapses under the force of its own gravity, creating a singularity – a point of infinite density and zero volume.
🧲 B. Can Magnetars Escape Black Holes? Understanding the Possibility
No, a magnetar cannot escape a black hole once it is inside the event horizon – the point of no return. This is because the gravitational force of a black hole is so strong that it overcomes even the immense magnetic field of a magnetar.
🌀 C. What happens when a magnetar gets too close to a black hole?
When a magnetar gets too close to a black hole, it will be stretched and compressed by tidal forces – the gravitational pull of the black hole that varies across the magnetar’s body. This process is known as spaghettification, and it will eventually tear the magnetar apart into a stream of subatomic particles. As the magnetar falls towards the black hole, it will also emit intense bursts of X-rays and gamma-rays due to the frictional heating caused by its interaction with the surrounding gas and dust.
X. Can a Magnetar Become a Black Hole? 🌟🕳️
A. How do Black Holes Form? 🕳️🌌
Black holes form when massive stars, at least 20 times more massive than the sun, run out of fuel and undergo a supernova explosion. The remaining core, which is incredibly dense, then collapses under the force of gravity and forms a singularity. The singularity is a point of infinite density and zero volume, surrounded by an event horizon, beyond which no light can escape.
B. Is it Possible for a Magnetar to Become a Black Hole? 🤔
It is possible for a magnetar to become a black hole, but it depends on the mass of the magnetar. If a magnetar has a mass less than three times the mass of the sun, it will not collapse into a black hole when it dies. Instead, it will gradually cool down over trillions of years until it becomes a cold, dark object called a black dwarf. However, if a magnetar has a mass greater than three times the mass of the sun, it could collapse into a black hole when it dies.
C. What Happens When a Magnetar Collapses? 💥🕳️
When a magnetar collapses, it undergoes a catastrophic implosion that compresses its mass into an incredibly small volume. If the mass is sufficient, this implosion can cause the formation of a black hole. The magnetar’s outer layers collapse inward at a velocity close to the speed of light, and the resulting explosion produces a burst of gamma rays that can be detected from Earth. The process of magnetar collapse into a black hole is a complex and poorly understood phenomenon that is the subject of ongoing research.
XI. Conclusion
A. What We’ve Learned About Magnetars📝?
Magnetars are a fascinating type of neutron star with an extremely strong magnetic field. They emit high-energy electromagnetic radiation, including X-rays and gamma rays, and have a number of unique characteristics. In this blog, we have explored what magnetars are, how they differ from black holes and pulsars, their effects on their surroundings and on Earth, and the current and future research on magnetars.
B. The Importance of Further Research on Magnetars💻🔬
Magnetars have the potential to provide valuable insights into the physics of extreme environments, and the study of magnetars can help us better understand the universe as a whole. By continuing research on magnetars, we can deepen our understanding of the magnetic fields and high-energy phenomena that occur in the universe, and develop new technologies and techniques for observing and studying these objects.
C. Final thoughts 💭
As we continue to learn more about magnetars, we will gain new insights into the fundamental nature of the universe and the processes that shape it. From the formation and evolution of stars and galaxies, to the fundamental physics that underlies the behavior of matter and energy, magnetars offer a unique window into the most extreme environments in the universe. By studying magnetars, we can unlock new discoveries and deepen our understanding of the cosmos, and take one step closer to understanding our place in the universe.
If you want to gain further knowledge about it, you can purchase the recommended book